Afrontando un diagnóstico
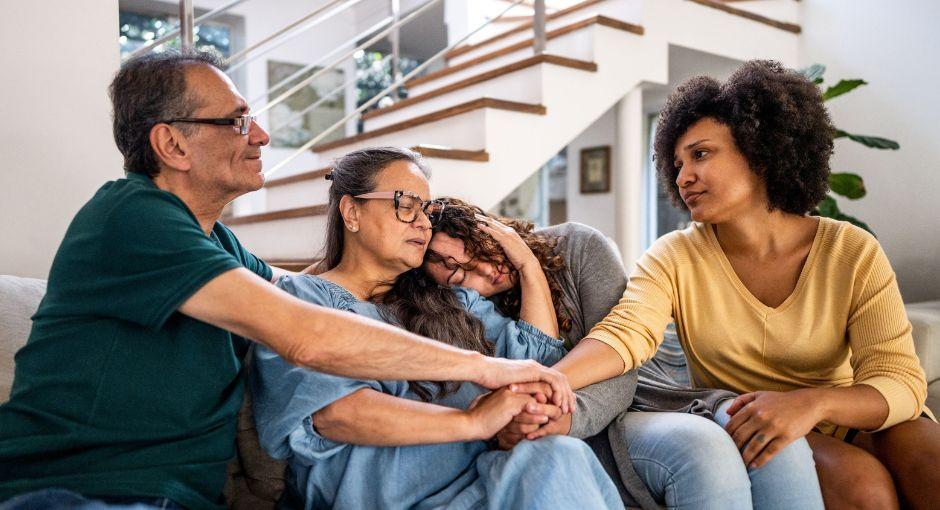
Cuando a usted o a un familiar le diagnostican la enfermedad de Parkinson (EP), experimentará una serie de emociones y pasará por varias etapas de adaptación a la enfermedad. A medida que la EP avance y traiga consigo nuevos desafíos, volverá a pasar por muchas de las emociones y etapas de adaptación. Cada individuo experimenta las etapas en su orden propio y a su propio ritmo.
Negación, incredulidad, shock
La negación puede ser un mecanismo de afrontamiento útil si le permite ignorar en gran medida los síntomas y seguir con su vida habitual. Sin embargo, si la negación lleva a negarse a tomar la medicación o a llegar al extremo de buscar múltiples segundas opiniones, puede indicar que la negación es una respuesta no saludable.
Esta respuesta puede prolongarse si los síntomas son leves o si no se realiza el diagnóstico correcto poco después de que aparezcan los síntomas.
Desánimo, en busca de una explicación
En esta fase, la gente busca alguna causa directa para los problemas de salud que experimenta. Puede que se la pase preguntándose "¿por qué a mí?" mientras busca algo o a alguien a quien culpar de las circunstancias no deseadas que han surgido en su vida.
Habilidades cambiantes, nuevos roles
Las personas con EP suelen necesitar más tiempo para realizar actividades debido a cambios en la coordinación de las manos, la rigidez muscular o la ralentización. Pueden surgir conflictos conforme se vuelva necesario revalorar quién es responsable de qué tareas en la familia y en el hogar.
Los cambios en las habilidades y el asumir nuevas funciones pueden causar frustración y trastornos emocionales, mientras que el estrés empeora los síntomas de la EP.
Cambio de identidad
En esta fase, puede que se dé cuenta de que la EP ha afectado su vida. Está dispuesto a hacer su parte para alcanzar su nivel óptimo de independencia y conectar con otros que comparten la misma condición para recibir educación y ánimos.
Recuerde que no está solo. Un millón de personas en los EE.UU. y 10 millones en todo el mundo viven con la EP. Estas cifras no toman en cuenta los casos de la EP no reportados, no diagnosticados o diagnosticados erróneamente. Con un diagnóstico y la libertad de aprender a su propio ritmo, puede empezar a comprender la EP, sus tratamientos y el papel que desempeñarán en su vida. Su diagnóstico puede ser el primer paso para tomar las riendas de su vida con la EP. ¿Cuáles son los siguientes pasos?
Escuche historias de personas con EP
Es habitual que muchos experimenten una amplia gama de emociones tras el diagnóstico, desde shock hasta enfado e incluso a veces una sensación de alivio al poder ponerle nombre a síntomas (quizás un pequeño temblor o debilidad) que no han tenido explicación o se han diagnosticado erróneamente durante años. Escuche a otras personas que hayan tenido una experiencia similar. Comience con Mi historia con la EP.
Encuentre recursos de ayuda
Línea de Ayuda
Llame a nuestra Línea de Ayuda o envíe un correo electrónico para obtener respuestas a sus preguntas: desde información acerca de la EP hasta derivaciones a especialistas cercanos.
¿Recién conociendo el Parkinson?
Ofrecemos recursos y asesoría a la comunidad para apoyarlos a usted y a su familia mientras comienzan a crear una mejor vida con el Parkinson.
Clases locales y atención experta
Utilice nuestra herramienta de búsqueda para encontrar centros médicos especializados en Parkinson, especialistas cercanos, su Chapter más próximo y recursos locales.
Página revisada por el Dr. Kevin Duque, investigador clínico en Neurología, División de Trastornos del Movimiento, en la University of Cincinnati.